北極圏メタンガス放散

北極圏メタンガス放散(ほっきょくけんメタンガスほうさん、Arctic methane emissions)は、北極の永久凍土地域の海洋および土壌からのメタンガスの放出をいう。北極地域には埋蔵天然ガスや海底のメタンクラスレート(メタンハイドレート)として、大量のメタンが蓄積されている。[1] メタン放散は長期にわたる自然現象だが永久凍土が地球温暖化により解凍すると、凍結していた大量のバイオマスのメタン発酵分解ならびにメタンクラスレートから大量のメタンが放出されることにより[2][3][4][5]、その量が増加する。[6] メタンは強力な温室効果ガスであるため一層温暖化を加速し正のフィードバックサイクルが生じ、その結果地球温暖化が急激かつ不可逆的に進行しうる。[7][8]

米国海洋大気庁の年次記録によると、2018年頃から大気中メタン濃度の増加が一貫して加速しており、2020年の増加は15.06 ppbで、1991年の14.05 ppbという以前の記録を上回った。2021年には18.34 ppbというさらに大きな増加が記録された。[10] 北極とこの最近の加速とを関連付ける証拠は今のところないが[11] 、これらの傾向は気候科学者を警戒させており、2022年のNature誌は危機的に速いと警告している。[12]
一方2021年の研究では、地球大気中のメタン濃度における北極圏の役割は過大評価されており、熱帯地域の役割が過小評価されているとしている。[13] この研究は、最近のメタン濃度の増加傾向の背後にあるのは熱帯湿地からのメタン排出である可能性を示唆し、この示唆は2022年の論文によって支持された。その2022年の論文では、2010年から2019年までの間に熱帯陸域の排出が大気中メタン濃度増加傾向の80%に関連しているとしている。[14] このような議論はあるものの、北極圏のメタン濃度増加傾向への影響が近い将来に増加する可能性は大きい。2022年の別の研究は、シベリアのツンドラ地域から大気中に温暖化に関連したメタンの排出が2004年以来増加しているという証拠を示した。[15]
原因と地球温暖化悪化に及ぼす度合い[編集]
永久凍土の解凍[編集]
北極地域における地球温暖化は、既存のメタンクラスレート貯蔵からのメタン放出と、バイオマスの発酵によるメタン生成を加速させる。[16] 水分が多いところではバイオマスが空気に晒されないためより低酸素すなわち嫌気的環境となる。バイオマスからのメタン生成には嫌気的環境が必要なため、古い埋蔵炭素からのメタン発生を遅くする。実際、2015年の研究によると、解凍され湿潤な嫌気的永久凍土地域からの累積メタン排出量は、好気的地域からの累積排出量の75-85%低かったと推定され、メタン排出はそれぞれの地域での二酸化炭素排出量の3-7%であった。これらは100年間を基準とした際の二酸化炭素排出の潜在的な気候への影響の25-45%を占め、好気的な永久凍土地域の解凍が全体としてはより大きな温暖化の影響を持つと結論づけられた。[17] しかしながら、2018年の別の研究では7年間の培養実験を行い、メタン生成微生物群集は嫌気的条件下で確立されると、好気性条件下でよりも多くの二酸化炭素当量を生成するようになった。したがって嫌気的永久凍土地域の解凍が全体的な温暖化に及ぼす影響は、以前まで考えられていたよりも大きいと結論された。[18]
メタン生成経路は嫌気的環境を必要とすることと、北極圏の湖などでしばしば観察されるメタンの気泡の出現とは関連している。[19][20] 氷が豊富な永久凍土の解凍によりできた湖(サーモカルスト湖)の堆積物で生成されたメタンのすべてが大気中に放散するわけではなく、水中または堆積物中で酸化される可能性もある。[21] しかし2022年の観測結果によれば、サーモカルスト湖内で生成されたメタンの少なくとも半分は大気中に放散している。[22] しばしば大量のメタン放散をもたらす別のプロセスは、永久凍土で安定化された斜面の浸食と崩壊である。[23] 2つの引き続くプロセス、すなわち斜面の崩壊(リトログレッシブ・ソー・スランプまたはRTS)とサーモカルスト湖の形成は、総じて急激な解凍によるもので、これらにより数日間のうちに大量の土壌が微生物呼吸にさらされる。これは、ほとんどの永久凍土環境で支配的な、かつて凍結していた土壌がセンチメートル単位で徐々に解凍されるのとは対照的である。この急速さは、"中間"シナリオの代表的濃度経路(RCP)4.5では70年以上は解凍から免れるとされていた3箇所の永久凍土が2019年に急激に解凍されたことで明白になった。[24] 2020年のシベリアの熱波の影響でさらに別の解凍も発生、北部のタイミル半島全体でRTSが82箇所から1404箇所と実に17倍も増加し、その結果土壌炭素の動員が28倍増加、半島全体で1平方メートルあたり年間平均11グラム(5ないし38グラム)もの炭素に達した。[25]
従来の永久凍土炭素フィードバック(PCF)モデリングは、急激な解凍をモデル化する難しさとメタン生成の速度に関する誤った仮定のために、緩慢な永久凍土の解凍のみを考慮してした。[26] しかし2018年の研究は、現地の観察、放射性炭素年代測定、およびサーモカルスト湖を考慮に入れるためのリモートセンシングを使用した結果、急激な解凍が進行しており2100年までに永久凍土からの炭素排出は2倍以上に増加することを明らかにした。[27] さらに2020年の研究では、持続的な加速排出のシナリオRCP 8.5の下では、2.5百万平方キロメートルにわたる急激な解凍の炭素排出が、それが占める全18百万平方キロメートルにわたる地表近くの永久凍土の徐々な解凍に等しい正のフィードバックを引き起こすと予測した。[26] その結果急激な解凍が2300年までに60から100ギガトンの放出炭素を追加し、[28] 単独の徐々な解凍と比較して炭素排出を約125-190%増加させる。[26][27]

しかし、解凍された永久凍土環境におけるメタン生成の速度と時間経過については議論がある。たとえば2017年の論文は、頻繁なサーモカルスト湖が存在する解凍湿原でさえ、メタン排出は10%未満が解凍された古い炭素に帰せられ、残りは新しい炭素源(バイオマス)の嫌気分解に起因しているとした。[30] 2018年のフォローアップの研究は、サーモカルスト湿地での急速な泥炭形成による炭素固定の増加が、メタンの増加放出を補償するだろうとさえ示唆した。[31] 2018年の別の論文では、サーモカルスト解凍の後、永久凍土からの排出は制限されているが、山火事の直後にはかなり増加すると示唆した。[32] 2022年の論文では、永久凍土解凍による湿原メタン排出が最初は非常に多いものの(平方メートルあたりのメタン82ミリグラム/日)、その湿原が成熟するにつれてほぼ3分の1に減少し、急激な解凍の数十年から百年後にはメタン排出が減少する可能性があるとした。[29]
2011年に行われた予備的分析では、永久凍土からのメタン排出が人為的排出の約15%に相当する可能性があるとした。[33] 2018年の展望記事では世界的な気温が約2℃上昇すると、永久凍土解凍が2100年までに世界の気温にさらに0.04-0.16℃を追加する可能性があるとしたが、[34] 急激な解凍は考慮に入っていない。IPCC第六次評価報告書によれば、永久凍土から放出される二酸化炭素とメタンは、気温上昇1°Cあたり14から1750億トンの二酸化炭素に相当すると推定された。比較を述べれば、2019年までの一年当たり二酸化炭素排出量は約400億トンであった。[35]:1237
急激な解凍を考慮に入れた永久凍土からの温暖化ガス排出の集合的な見積もり(二酸化炭素・メタン合算)は2021年に発表され、それによると2100年までの数値は、1.5℃の温暖化の場合には150-2000億トンの二酸化炭素換算量、2℃の場合には220-3000億トン、そして温暖化が4℃を超える場合には400-5000億トンであり、これは1.5℃目標内に留まるための残りのカーボンバジェット全部をこれだけで使い切ることを意味する。[36] 同じ著者はまた別の論文で、温暖化による永久凍土からの排出の増加が、現在進行中の二酸化炭素排出と組み合わされた場合、パリ協定1.5℃の温暖化の超過を回避することは不可能と示唆した。[37] 1.5℃温暖化が守れなかった場合に地球規模で何が起こるかを総合的に研究した2022年のサイエンス誌の論文によれば、急激な永久凍土解凍は徐々な解凍の率に50%を追加し、3-6℃の温暖化の範囲で(約4℃が最も可能性が高い)永久凍土地域の大規模な崩壊は不可逆になり、約50年間(範囲は10年から300年)で175から3500億トンの二酸化炭素換算量の排出、0.2-0.4℃の温暖化が追加される。[38][39]
北極圏海氷の減少[編集]
2015年の研究によれば北極海氷の減少は北極ツンドラからのメタン排出を促進しており、2005年から2010年の排出量は、海氷が1981年から1990年の水準にあった場合よりも約170万トン多いと結論した。[40] [41]
海底や永久凍土深層のメタンクラスレートの崩壊[編集]

海温の上昇が引き金となって、海底に埋まっているメタンクラスレートや、海底の永久凍土内のメタンが放出され、メタン自体の強力な温室効果ガス効果によりさらなる気温上昇とメタンクラスレートの不安定化をもたらし、一度始まると急激かつ不可逆的な温暖化の暴走プロセスを開始するとされる仮説の俗称を(メタン)クラスレート銃の仮説という。[42] この仮説では、海洋の上部中間層の変化が、断続的にメタンクラスレートを上部大陸スロープに蓄積し、時折メタンを放出する温度変動を引き起こしたとしている。メタンの大気寿命は約12年であるものの二酸化炭素の約25倍の温暖化効力のせいで、放出メタンの地球温暖化ポテンシャルは20年間で二酸化炭素の72倍、100年間で25倍(エアロゾルとの相互作用を考慮すると33倍)と見積もられている。[43]

この仮説には議論があり[44] これは千年の時間尺度での現象の説明には有用でも[45][46] 近い将来の気候変動に関連するとは考えられていなかった。[47] 殆どのメタンクラスレートは地上の温暖化に迅速に反応するにはあまりに深い地下に堆積している[48]からである。IPCC第六次評価報告書も「この世紀中にガスクラスレート(主にメタン)が陸上の永久凍土深層や海底から検出可能な逸脱を引き起こす可能性は非常に低い」と述べている。[49] しかし北極圏の一部のメタンクラスレートは他の場所よりもはるかに浅いところにあり温暖化に対してより脆弱な可能性がある。カナダのビョーフォート海にある大陸斜面にあるメタンクラスレートは海底の小さな円錐状の丘のあるエリアに位置しており、海面からわずか290メートルしかない。[50] 東シベリアの北極圏大陸棚は平均して水深わずか45メートルであり、海底以下には海底の永久凍土層によって封じられた状態でハイドレートの堆積物が存在すると考えられている。[51][52]
タリクやピンゴのような永久凍土の部分構造は、北極圏大陸棚内に封鎖されているメタンが温暖化により地表に排出するホットスポットとして機能しうる。[53][54][55] シャホワら(2008)によると、東シベリアの北極圏大陸棚の永久凍土の下には少なくとも1,400ギガトンの炭素がメタンおよびメタンクラスレートとして閉じ込められており、その領域の5-10%がタリクを通じて突き破られる可能性がある。[56] この量のメタン放散が起こると大気中のメタン含有量は一気に12倍となり、2008年時点での二酸化炭素レベルが倍になるのと同等の温室効果を引き起こしうる。[57] 2012年の研究でも東シベリア北極圏大陸棚のメタンクラスレートについて同様な結論を述べている。[58]

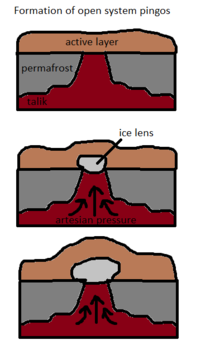
シベリア北極圏で行われ2007年に発表された研究によれば、年間数百万トンのスケールでメタンが放出され、これは以前の年間約50万トンの見積もりより遥かに大きい。[59] これは明らかに永久凍土の穴を通じてであり、[55] 一部の地域では通常の濃度の100倍に達することがある。[60][61] 過剰なメタンは、レナ川の河口とラプテフ海と東シベリア海の境界にある局所的なホットスポットで検出されている。2013年までに同じ研究チームは、複数のソナー観測を使用して、海底の永久凍土から海洋中に噴出する気泡の密度から、東シベリア北極圏大陸棚では1平方メートル当たり100-630 ミリグラムのメタンが毎日海洋に放出されていることを発見した。[62] 2020年1月に北極で複数の船で行われた観測では、東シベリア北極圏大陸棚からのメタン放出量を年間約302万トンと見積もった。[63]
2017年6月、トロムソ大学の極地ガスハイドレート環境および気候センターの科学者たちが、メタンクラスレートに起因するとされる爆発的な噴出によって形成された、直径約300メートル深さ最大30メートルの海洋クレーター100箇所以上について発表した。これらは今もベアント海周辺でメタンを噴出しており、メタンを蓄積している既存の隆起が同じ運命をたどる可能性があるとした。[64]
2021年タイミル半島とその周辺域で、その1年前2020年夏の気温上昇により永久凍土域の石灰岩構造物中に封じられていたメタンクラスレートが不安定化し、そのわずか1年の間で大気中メタン濃度が100 ppbも上昇した。[65] その地域は湿地土壌はわずかしかなく、硬い石灰岩がメタンの放出源であることは驚嘆に値する。このことはシベリアの気温上昇は湿地の永久凍土の解凍から見積もられるよりも遥かに多量のメタンを放散しうることを示している。[66] 2022年の論文によると、シベリア・レナ川流域永久凍土地帯の6月の気温が1年前に比べて0.3 ± 0.1 °C上がると、6・7月のメタン排出量は1.9 ± 0.7%増加した。温暖化に伴う初夏の気温上昇はメタン排出量の著しい増加を引き起こすことが明白である。[67]
対策[編集]
メタン放散の緩和対策は2020年代に実施されれば、北極の海氷解凍を防止できる可能性があるとする研究がある。この研究によれば二酸化炭素排出量のみの緩和では不十分でメタン排出をともに抑制することが有効としている。[68]
メタンは化石燃料である。メタンを回収してフレア燃焼し二酸化炭素として放出する対策が試みられている。もちろん二酸化炭素も温暖化ガスであるがその温暖化係数はメタンの25分の1で済む。問題となるのは大気中に放散されたメタンは希釈され回収が困難なことである。2012年時点で既存の技術はメタン0.1%(1000ppm)以上でのみ適用可能であり、これは自然放散量の数百倍でメタンが地中から気泡で発生しているようなホットスポットに限られる。[69] ARPA-Eは、2021年から2023年にかけて、遠隔地で放散しているメタンを燃焼させるための「スマート・マイクロフレア・フリート」を開発する研究プロジェクトに資金提供した。[70][71][72]
関連項目[編集]
- Atmospheric methane
- Arctic sea ice decline
- Climate change mitigation
- Effects of climate change
- Greenhouse gas emissions
- Arctic dipole anomaly
- Arctic peat fires
- Clathrate hydrate
- Climate change in Antarctica
- Effects of climate change
- Methane clathrate
引用[編集]
- ^ Bloom, A. A.; Palmer, P. I.; Fraser, A.; Reay, D. S.; Frankenberg, C. (2010). “Large-Scale Controls of Methanogenesis Inferred from Methane and Gravity Spaceborne Data”. Science 327 (5963): 322–325. Bibcode: 2010Sci...327..322B. doi:10.1126/science.1175176. PMID 20075250. オリジナルの2018-07-22時点におけるアーカイブ。 2019年12月3日閲覧。.
- ^ Zimov, Sa; Schuur, Ea; Chapin, Fs 3Rd (Jun 2006). “Climate change. Permafrost and the global carbon budget”. Science 312 (5780): 1612–3. doi:10.1126/science.1128908. ISSN 0036-8075. PMID 16778046 .
- ^ Shakhova, Natalia (2005). “The distribution of methane on the Siberian Arctic shelves: Implications for the marine methane cycle”. Geophysical Research Letters 32 (9): L09601. Bibcode: 2005GeoRL..32.9601S. doi:10.1029/2005GL022751.
- ^ Shakhova, Natalia; Semiletov, Igor (2007). “Methane release and coastal environment in the East Siberian Arctic shelf”. Journal of Marine Systems 66 (1–4): 227–243. Bibcode: 2007JMS....66..227S. doi:10.1016/j.jmarsys.2006.06.006 .
- ^ Sayedi, Sayedeh Sara; Abbott, Benjamin W; Thornton, Brett F; Frederick, Jennifer M; Vonk, Jorien E; Overduin, Paul; Schädel, Christina; Schuur, Edward A G et al. (2020-12-01). “Subsea permafrost carbon stocks and climate change sensitivity estimated by expert assessment”. Environmental Research Letters 15 (12): B027-08. Bibcode: 2020AGUFMB027...08S. doi:10.1088/1748-9326/abcc29. hdl:10852/83674. ISSN 1748-9326.
- ^ Walter, K. M.; Chanton, J. P.; Chapin, F. S.; Schuur, E. A. G.; Zimov, S. A. (2008). “Methane production and bubble emissions from arctic lakes: Isotopic implications for source pathways and ages”. Journal of Geophysical Research 113: G00A08. Bibcode: 2008JGRG..11300A08W. doi:10.1029/2007JG000569 .
- ^ Cheng, Chin-Hsien; Redfern, Simon A. T. (23 June 2022). “Impact of interannual and multidecadal trends on methane-climate feedbacks and sensitivity”. Nature Communications 13 (1): 3592. Bibcode: 2022NatCo..13.3592C. doi:10.1038/s41467-022-31345-w. PMC 9226131. PMID 35739128 .
- ^ Christensen, Torben Røjle; Arora, Vivek K.; Gauss, Michael; Höglund-Isaksson, Lena; Parmentier, Frans-Jan W. (4 February 2019). “Tracing the climate signal: mitigation of anthropogenic methane emissions can outweigh a large Arctic natural emission increase”. Scientific Reports 9 (1): 1146. doi:10.1038/s41598-018-37719-9. PMC 6362017. PMID 30718695 .
- ^ Saunois, M.; Stavert, A.R.; Poulter, B. (July 15, 2020). “The Global Methane Budget 2000–2017” (英語). Earth System Science Data (ESSD) 12 (3): 1561–1623. Bibcode: 2020ESSD...12.1561S. doi:10.5194/essd-12-1561-2020. ISSN 1866-3508 2020年8月28日閲覧。.
- ^ “Trends in Atmospheric Methane”. NOAA. 2022年10月14日閲覧。
- ^ “Increasing anthropogenic methane emissions arise equally from agricultural and fossil fuel sources”. Environmental Research Letters 15 (7): 071002. (15 July 2020). Bibcode: 2020ERL....15g1002J. doi:10.1088/1748-9326/ab9ed2.
- ^ “Scientists raise alarm over 'dangerously fast' growth in atmospheric methane”. Nature. (8 February 2022) 2022年10月14日閲覧。.
- ^ “Improved Constraints on Global Methane Emissions and Sinks Using δ13C-CH4”. Global Biogeochemical Cycles 35 (6): e2021GB007000. (8 May 2021). Bibcode: 2021GBioC..3507000L. doi:10.1029/2021GB007000. PMC 8244052. PMID 34219915 .
- ^ Feng, Liang; Palmer, Paul I.; Zhu, Sihong; Parker, Robert J.; Liu, Yi (16 March 2022). “Tropical methane emissions explain large fraction of recent changes in global atmospheric methane growth rate” (英語). Nature Communications 13 (1): 1378. Bibcode: 2022NatCo..13.1378F. doi:10.1038/s41467-022-28989-z. PMC 8927109. PMID 35297408 .
- ^ Rößger, Norman; Sachs, Torsten; Wille, Christian; Boike, Julia; Kutzbach, Lars (27 October 2022). “Seasonal increase of methane emissions linked to warming in Siberian tundra”. Nature Climate Change 12 (11): 1031–1036. Bibcode: 2022NatCC..12.1031R. doi:10.1038/s41558-022-01512-4 2023年1月21日閲覧。.
- ^ Walter, K. M.; Chanton, J. P.; Chapin, F. S.; Schuur, E. A. G.; Zimov, S. A. (2008). “Methane production and bubble emissions from arctic lakes: Isotopic implications for source pathways and ages”. Journal of Geophysical Research 113: G00A08. Bibcode: 2008JGRG..11300A08W. doi:10.1029/2007JG000569.
- ^ Schuur, E. A. G.; McGuire, A. D.; Schädel, C.; Grosse, G.; Harden, J. W. (9 April 2015). “Climate change and the permafrost carbon feedback”. Nature 520 (7546): 171–179. Bibcode: 2015Natur.520..171S. doi:10.1038/nature14338. PMID 25855454 .
- ^ Pfeiffer, Eva-Maria; Grigoriev, Mikhail N.; Liebner, Susanne; Beer, Christian; Knoblauch, Christian (April 2018). “Methane production as key to the greenhouse gas budget of thawing permafrost”. Nature Climate Change 8 (4): 309–312. Bibcode: 2018NatCC...8..309K. doi:10.1038/s41558-018-0095-z. ISSN 1758-6798 .
- ^ Walter, KM; Zimov, SA; Chanton, JP; Verbyla, D et al. (7 September 2006). “Methane bubbling from Siberian thaw lakes as a positive feedback to climate warming”. Nature 443 (7107): 71–75. Bibcode: 2006Natur.443...71W. doi:10.1038/nature05040. PMID 16957728.
- ^ Gillis, Justin (2011年12月16日). “As Permafrost Thaws, Scientists Study the Risks”. The New York Times 2011年12月17日閲覧。
- ^ Vigderovich, Hanni; Eckert, Werner; Elul, Michal; Rubin-Blum, Maxim; Elvert, Marcus; Sivan, Orit; Czimczik, C. I. (2 May 2022). “Long-term incubations provide insight into the mechanisms of anaerobic oxidation of methane in methanogenic lake sediments”. Biogeosciences 19 (8). Bibcode: 2022GeoRL..4997347P. doi:10.1029/2021GL097347 .
- ^ Pellerin, André; Lotem, Noam; Anthony, Katey Walter; Russak, Efrat Eliani; Hasson, Nicholas; Røy, Hans; Chanton, Jeffrey P.; Sivan, Orit (4 March 2022). “Methane production controls in a young thermokarst lake formed by abrupt permafrost thaw”. Global Change Biology 28 (10): 3206–3221. doi:10.1111/gcb.16151. PMC 9310722. PMID 35243729 .
- ^ Turetsky, Merritt R. (2019-04-30). “Permafrost collapse is accelerating carbon release”. Nature 569 (7754): 32–34. Bibcode: 2019Natur.569...32T. doi:10.1038/d41586-019-01313-4. PMID 31040419.
- ^ “Scientists shocked by Arctic permafrost thawing 70 years sooner than predicted” (英語). The Guardian. (2019年6月18日). ISSN 0261-3077 2019年7月2日閲覧。
- ^ Bernhard, Philipp; Zwieback, Simon; Hajnsek, Irena (2 May 2022). “Accelerated mobilization of organic carbon from retrogressive thaw slumps on the northern Taymyr Peninsula”. The Cryosphere 16 (7): 2819–2835. Bibcode: 2022TCry...16.2819B. doi:10.5194/tc-16-2819-2022 .
- ^ a b c Turetsky, Merritt R.; Abbott, Benjamin W.; Jones, Miriam C.; Anthony, Katey Walter; Olefeldt, David; Schuur, Edward A. G.; Grosse, Guido; Kuhry, Peter et al. (February 2020). “Carbon release through abrupt permafrost thaw”. Nature Geoscience 13 (2): 138–143. Bibcode: 2020NatGe..13..138T. doi:10.1038/s41561-019-0526-0. ISSN 1752-0894.
- ^ a b Walter Anthony, Katey; Schneider von Deimling, Thomas; Nitze, Ingmar; Frolking, Steve; Emond, Abraham; Daanen, Ronald; Anthony, Peter; Lindgren, Prajna et al. (2018-08-15). “21st-century modeled permafrost carbon emissions accelerated by abrupt thaw beneath lakes”. Nature Communications 9 (1): 3262. Bibcode: 2018NatCo...9.3262W. doi:10.1038/s41467-018-05738-9. ISSN 2041-1723. PMC 6093858. PMID 30111815 .
- ^ “Permafrost collapse is accelerating carbon release”. Nature 569 (7754): 32–34. (May 2019). Bibcode: 2019Natur.569...32T. doi:10.1038/d41586-019-01313-4. PMID 31040419.
- ^ a b >Heffernan, Liam; Cavaco, Maria A.; Bhatia, Maya P.; Estop-Aragonés, Cristian; Knorr, Klaus-Holger; Olefeldt, David (24 June 2022). “High peatland methane emissions following permafrost thaw: enhanced acetoclastic methanogenesis during early successional stages”. Biogeosciences 19 (8): 3051–3071. Bibcode: 2022BGeo...19.3051H. doi:10.5194/bg-19-3051-2022 .
- ^ Cooper, M.; Estop-Aragonés, C.; Fisher, J. (26 June 2017). “Limited contribution of permafrost carbon to methane release from thawing peatlands”. Nature Climate Change 7 (7): 507–511. Bibcode: 2017NatCC...7..507C. doi:10.1038/nclimate3328 .
- ^ Estop-Aragonés, Cristian; Cooper, Mark D.A.; Fisher, James P. (March 2018). “Limited release of previously-frozen C and increased new peat formation after thaw in permafrost peatlands”. Soil Biology and Biochemistry 118: 115–129. doi:10.1016/j.soilbio.2017.12.010.
- ^ Estop-Aragonés, Cristian (13 August 2018). “Respiration of aged soil carbon during fall in permafrost peatlands enhanced by active layer deepening following wildfire but limited following thermokarst”. Environmental Research Letters 13 (8): 085002. Bibcode: 2018ERL....13h5002E. doi:10.1088/1748-9326/aad5f0.
- ^ Gillis, Justin (2011年12月16日). “As Permafrost Thaws, Scientists Study the Risks”. The New York Times. オリジナルの2017年5月19日時点におけるアーカイブ。 2017年2月11日閲覧。
- ^ Schellnhuber, Hans Joachim; Winkelmann, Ricarda; Scheffer, Marten; Lade, Steven J.; Fetzer, Ingo; Donges, Jonathan F.; Crucifix, Michel; Cornell, Sarah E. et al. (2018). “Trajectories of the Earth System in the Anthropocene”. Proceedings of the National Academy of Sciences 115 (33): 8252–8259. Bibcode: 2018PNAS..115.8252S. doi:10.1073/pnas.1810141115. ISSN 0027-8424. PMC 6099852. PMID 30082409 .
- ^ Intergovernmental Panel on Climate Change (2023-07-06). Climate Change 2021 – The Physical Science Basis: Working Group I Contribution to the Sixth Assessment Report of the Intergovernmental Panel on Climate Change (1 ed.). Cambridge University Press. doi:10.1017/9781009157896.011.. ISBN 978-1-009-15789-6
- ^ “Carbon Emissions from Permafrost” (英語). 50x30 (2021年). 2022年10月8日閲覧。
- ^ Natali, Susan M.; Holdren, John P.; Rogers, Brendan M.; Treharne, Rachael; Duffy, Philip B.; Pomerance, Rafe; MacDonald, Erin (10 December 2020). “Permafrost carbon feedbacks threaten global climate goals”. PNAS 118 (21). doi:10.1073/pnas.2100163118. PMC 8166174. PMID 34001617 .
- ^ Armstrong McKay, David; Abrams, Jesse; Winkelmann, Ricarda; Sakschewski, Boris; Loriani, Sina; Fetzer, Ingo; Cornell, Sarah; Rockström, Johan et al. (9 September 2022). “Exceeding 1.5°C global warming could trigger multiple climate tipping points” (英語). Science 377 (6611): eabn7950. doi:10.1126/science.abn7950. hdl:10871/131584. ISSN 0036-8075. PMID 36074831 .
- ^ Armstrong McKay, David (2022年9月9日). “Exceeding 1.5°C global warming could trigger multiple climate tipping points – paper explainer” (英語). climatetippingpoints.info. 2022年10月2日閲覧。
- ^ Parmentier, Frans-Jan W.; Zhang, Wenxin; Mi, Yanjiao; Zhu, Xudong; van Huissteden, Jacobus; J. Hayes, Daniel; Zhuang, Qianlai; Christensen, Torben R. et al. (25 July 2015). “Rising methane emissions from northern wetlands associated with sea ice decline”. Geophysical Research Letters 42 (17): 7214–7222. Bibcode: 2015GeoRL..42.7214P. doi:10.1002/2015GL065013. PMC 5014133. PMID 27667870 .
- ^ “Melting Arctic sea ice accelerates methane emissions”. ScienceDaily (2015年). 2019年6月8日時点のオリジナルよりアーカイブ。2018年3月9日閲覧。
- ^ 『Methane Hydrates in Quaternary Climate Change: The Clathrate Gun Hypothesis』John Wiley & Sons, Inc.、2003年1月1日。
- ^ Shindell, Drew T.; Faluvegi, Greg; Koch, Dorothy M.; Schmidt, Gavin A.; Unger, Nadine; Bauer, Susanne E. (2009). “Improved attribution of climate forcing to emissions”. Science 326 (5953): 716–718. Bibcode: 2009Sci...326..716S. doi:10.1126/science.1174760. PMID 19900930 .
- ^ Maslin, M; Owen, M; Betts, R; Day, S; Dunkley Jones, T; Ridgwell, A (2010-05-28). “Gas hydrates: past and future geohazard?” (英語). Philosophical Transactions of the Royal Society A: Mathematical, Physical and Engineering Sciences 368 (1919): 2369–2393. Bibcode: 2010RSPTA.368.2369M. doi:10.1098/rsta.2010.0065. ISSN 1364-503X. PMID 20403833.
- ^ Archer, David; Buffett, Bruce (2005). “Time-dependent response of the global ocean clathrate reservoir to climatic and anthropogenic forcing”. Geochemistry, Geophysics, Geosystems 6 (3): 1–13. Bibcode: 2005GGG.....603002A. doi:10.1029/2004GC000854. オリジナルの2009-07-09時点におけるアーカイブ。 2009年5月15日閲覧。.
- ^ Schellnhuber, Hans Joachim; Winkelmann, Ricarda; Scheffer, Marten; Lade, Steven J.; Fetzer, Ingo; Donges, Jonathan F.; Crucifix, Michel; Cornell, Sarah E. et al. (2018). “Trajectories of the Earth System in the Anthropocene”. Proceedings of the National Academy of Sciences 115 (33): 8252–8259. Bibcode: 2018PNAS..115.8252S. doi:10.1073/pnas.1810141115. ISSN 0027-8424. PMC 6099852. PMID 30082409 .
- ^ Archer, D. (2007). “Methane hydrate stability and anthropogenic climate change”. Biogeosciences 4 (4): 521–544. Bibcode: 2007BGeo....4..521A. doi:10.5194/bg-4-521-2007 . See also blog summary Archived 2007-04-15 at the Wayback Machine..
- ^ Archer, D.; Buffett, B. (2005). “Time-dependent response of the global ocean clathrate reservoir to climatic and anthropogenic forcing”. Geochemistry, Geophysics, Geosystems 6 (3): Q03002. Bibcode: 2005GGG.....603002A. doi:10.1029/2004GC000854 .
- ^ Fox-Kemper, B.; Hewitt, H.T.; Xiao, C.; Aðalgeirsdóttir, G.; Drijfhout, S.S.; Edwards, T.L.; Golledge, N.R.; Hemer, M. et al. (2021). Masson-Delmotte, V.; Zhai, P.; Pirani, A. et al.. eds. “Chapter 5: Global Carbon and other Biogeochemical Cycles and Feedbacks”. Climate Change 2021: The Physical Science Basis. Contribution of Working Group I to the Sixth Assessment Report of the Intergovernmental Panel on Climate Change (Cambridge University Press, Cambridge, UK and New York, NY, USA): 5. doi:10.1017/9781009157896.011 .
- ^ Corbyn, Zoë (December 7, 2012). “Locked greenhouse gas in Arctic sea may be 'climate canary'”. Nature. doi:10.1038/nature.2012.11988 2014年4月12日閲覧。.
- ^ Shakhova, N.; Semiletov, I.; Panteleev, G. (2005). “The distribution of methane on the Siberian Arctic shelves: Implications for the marine methane cycle”. Geophysical Research Letters 32 (9): L09601. Bibcode: 2005GeoRL..32.9601S. doi:10.1029/2005GL022751.
- ^ “Arctic methane outgassing on the E Siberian Shelf part 1 - the background”. SkepticalScience (2012年). 2023年12月29日閲覧。
- ^ “Climate-Hydrate Interactions”. USGS (2013年1月14日). 2023年12月29日閲覧。
- ^ “Methane release from the East Siberian Arctic Shelf and the Potential for Abrupt Climate Change” (2010年11月30日). 2014年4月12日閲覧。
- ^ a b "Methane bubbling through seafloor creates undersea hills" (Press release). Monterey Bay Aquarium Research Institute. 5 February 2007. 2008年10月11日時点のオリジナルよりアーカイブ。
- ^ Shakhova, N.; Semiletov, I.; Salyuk, A.; Kosmach, D. (2008). “Anomalies of methane in the atmosphere over the East Siberian shelf: Is there any sign of methane leakage from shallow shelf hydrates?”. Geophysical Research Abstracts 10: 01526. オリジナルの2012-12-22時点におけるアーカイブ。 2008年9月25日閲覧。.
- ^ Mrasek, Volker (2008年4月17日). “A Storehouse of Greenhouse Gases Is Opening in Siberia”. Spiegel International Online . "The Russian scientists have estimated what might happen when this Siberian permafrost-seal thaws completely and all the stored gas escapes. They believe the methane content of the planet's atmosphere would increase twelvefold."
- ^ Sergienko, V. I. (September 2012). “The Degradation of Submarine Permafrost and the Destruction of Hydrates on the Shelf of East Arctic Seas as a Potential Cause of the 'Methane Catastrophe': Some Results of Integrated Studies in 2011” (PDF). Doklady Earth Sciences 446 (1): 1132–1137. Bibcode: 2012DokES.446.1132S. doi:10.1134/S1028334X12080144. ISSN 1028-334X .
- ^ Shakhova, N.; Semiletov, I.; Salyuk, A.; Kosmach, D.; Bel'cheva, N. (2007). “Methane release on the Arctic East Siberian shelf”. Geophysical Research Abstracts 9: 01071 .
- ^ Connor, Steve (2008年9月23日). “Exclusive: The methane time bomb”. The Independent. 2008年10月3日閲覧。
- ^ Connor, Steve (2008年9月25日). “Hundreds of methane 'plumes' discovered”. The Independent. 2008年10月3日閲覧。
- ^ Shakhova, Natalia; Semiletov, Igor; Leifer, Ira; Sergienko, Valentin; Salyuk, Anatoly; Kosmach, Denis; Chernykh, Denis; Stubbs, Chris et al. (24 November 2013). “Ebullition and storm-induced methane release from the East Siberian Arctic Shelf”. Nature 7 (1): 64–70. Bibcode: 2014NatGe...7...64S. doi:10.1038/ngeo2007 .
- ^ Thornton, Brett F.; Prytherch, John; Andersson, Kristian; Brooks, Ian M.; Salisbury, Dominic; Tjernström, Michael; Crill, Patrick M. (29 January 2020). “Shipborne eddy covariance observations of methane fluxes constrain Arctic sea emissions”. Science Advances 6 (5): eaay7934. Bibcode: 2020SciA....6.7934T. doi:10.1126/sciadv.aay7934. PMC 6989137. PMID 32064354 .
- ^ “Like 'champagne bottles being opened': Scientists document an ancient Arctic methane explosion”. The Washington Post. (2017年6月1日)
- ^ Froitzheim, Nikolaus; Majka, Jaroslaw; Zastrozhnov, Dmitry (2021-08-10). “Methane release from carbonate rock formations in the Siberian permafrost area during and after the 2020 heat wave” (英語). Proceedings of the National Academy of Sciences 118 (32). doi:10.1073/pnas.2107632118. ISSN 0027-8424. PMC PMC8364203. PMID 34341110 .
- ^ Mufson, Steven (2021年8月3日). “Scientists expected thawing wetlands in Siberia’s permafrost. What they found is ‘much more dangerous.’” (英語). Washington Post. ISSN 0190-8286 2024年1月2日閲覧。
- ^ Rößger, Norman; Sachs, Torsten; Wille, Christian; Boike, Julia; Kutzbach, Lars (2022-11). “Seasonal increase of methane emissions linked to warming in Siberian tundra” (英語). Nature Climate Change 12 (11): 1031–1036. doi:10.1038/s41558-022-01512-4. ISSN 1758-6798 .
- ^ Sun, Tianyi; Ocko, Ilissa B; Hamburg, Steven P (2022-03-15). “The value of early methane mitigation in preserving Arctic summer sea ice” (英語). Environmental Research Letters 17 (4): 044001. Bibcode: 2022ERL....17d4001S. doi:10.1088/1748-9326/ac4f10. ISSN 1748-9326.
- ^ Stolaroff, Joshuah K.; Bhattacharyya, Subarna; Smith, Clara A.; Bourcier, William L.; Cameron-Smith, Philip J.; Aines, Roger D. (2012-06-19). “Review of Methane Mitigation Technologies with Application to Rapid Release of Methane from the Arctic” (英語). Environmental Science & Technology 46 (12): 6455–6469. Bibcode: 2012EnST...46.6455S. doi:10.1021/es204686w. ISSN 0013-936X. OSTI 1773262. PMID 22594483 .
- ^ “Frost Methane Labs: Design of Smart Micro-Flare Fleet to Mitigate Distributed Methane Emissions”. ARPA-E. 2022年7月24日閲覧。
- ^ Herman, Ari (2019年8月26日). “A Startup to Save All Startups: Mitigating Arctic Methane Release” (英語). The LegoBox Travelogue. 2022年7月24日閲覧。
- ^ “Home” (英語). Frost Methane Labs (2021年). 2022年7月24日閲覧。
参考資料[編集]
- Arctic permafrost is thawing fast. That affects us all. National Geographic, 2019
- Why the Arctic is smouldering, BBC Future, by Zoe Cormier, 2019